RESEARCH
CeeD particularly focuses on the implication of the muscle, liver, adipose tissue and kidneys in glucose homeostasis but also on the complications on these organs generated by diabetes.
Discover
Science for all (french version)
Discover a digest explanation about our research on our large audience campaign's website : Vaincre le diabète (Defeat diabetes) /
Retrouvez une présentation de nos travaux de recherche en français sur le site de notre initiative grand public : Vaincre le diabète
Integrative physiology: organ crosstalk
CeeD focuses on the hidden face of an iceberg, new interactions in this crosstalk communication between the different insulin-sensitive organs, which are discovered every day, highlighting the complexity of diabetes.
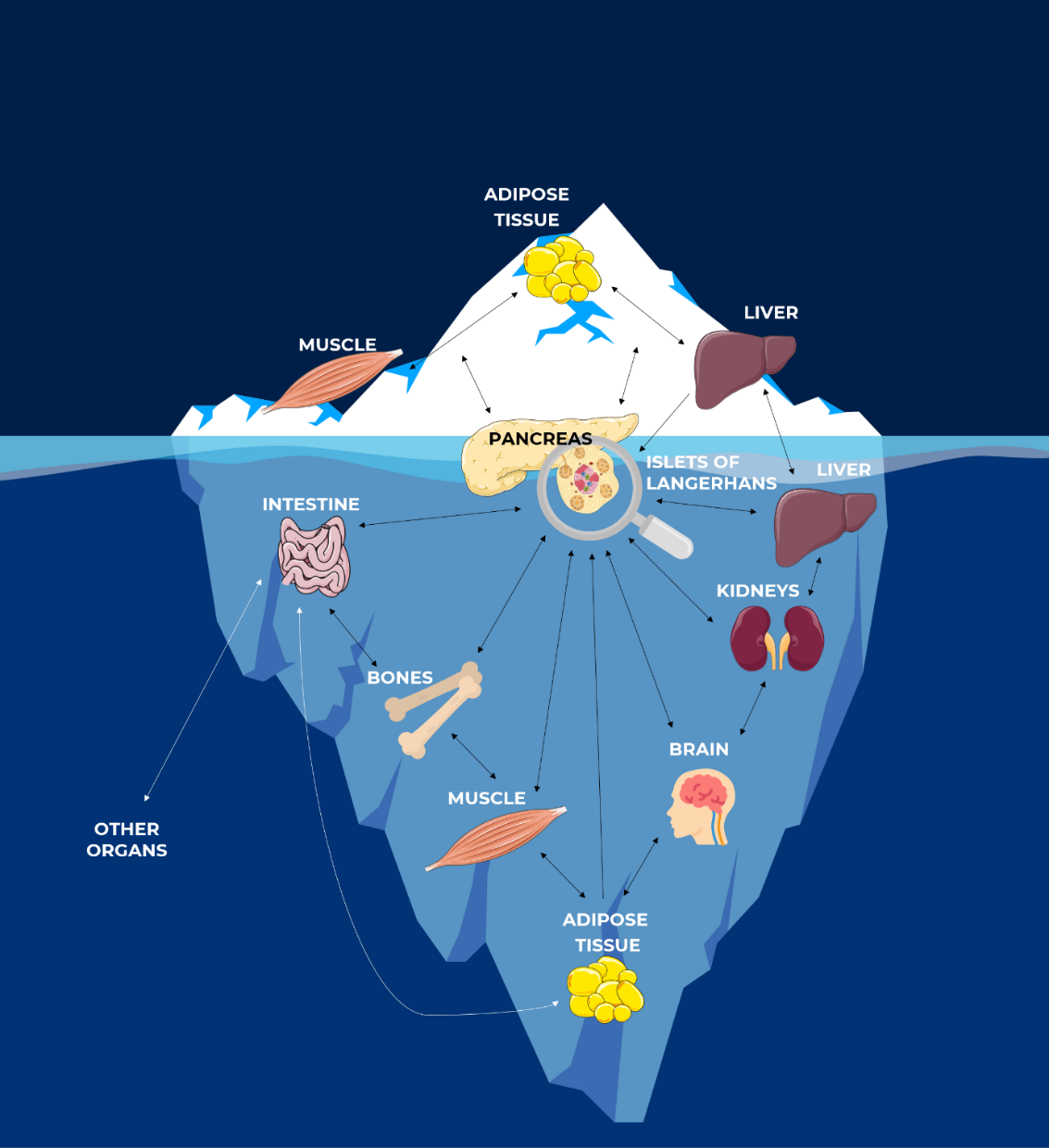
The Hidden Face of Iceberg: Crosstalk Communications Between Islets Cells and Insulin Target Tissue ©CeeD
#1. Type-1 diabetes and islet transplantation
Type 1 diabetes (T1D) is an autoimmune disease inducing the destruction of pancreatic β cells and hyperglycemia. One of the therapies proposed for patients with T1D is the injection of pancreatic islets into the liver [8], [9]. Islet transplantation is a promising strategy as it ensures:
the stabilization
of glucose metabolism
significant decrease in severe hypoglycemic episodes
the normalization of glycated hemoglobin levels
the delay or attenuation of diabetes complication [9]–[11]
Islet transplantation process
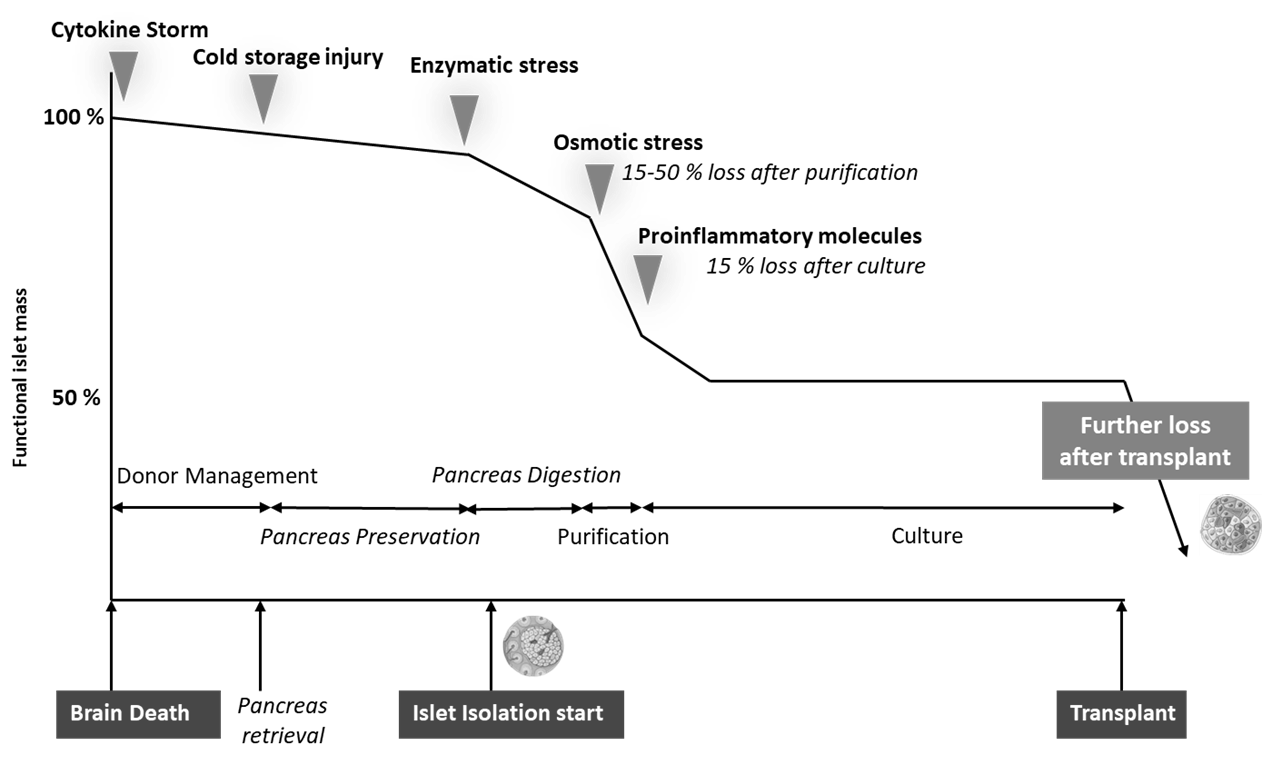
Moreover, this therapeutic approach is already approved and reimbursable through various national health systems in several countries, including Canada, Australia, the United Kingdom, Switzerland, Italy and France [12].
However, this therapy comes up against many obstacles, which still make it difficult to offer to a large number of diabetic patients. Indeed, throughout the entire transplant procedure, meaning from the pancreas donor to the isolation of the pancreatic islets and until the post-transplantation period, the quantity of functional islets is decreasing.
This loss can be explained by:
- the induction of cell death mechanisms due to the stress induced all along the islet transplantation process
- the hypoxia phenomenon during islet isolation
- the time of revascularization too long after islet transplantation in the hepatic portal vein
- Instant blood-mediated inflammatory reactions (IBMIR) and the secretion of pro-inflammatory cytokines
- rejection reactions
- the excessively long period of islets revascularization post-transplantation [9].
Transplanted islet destruction
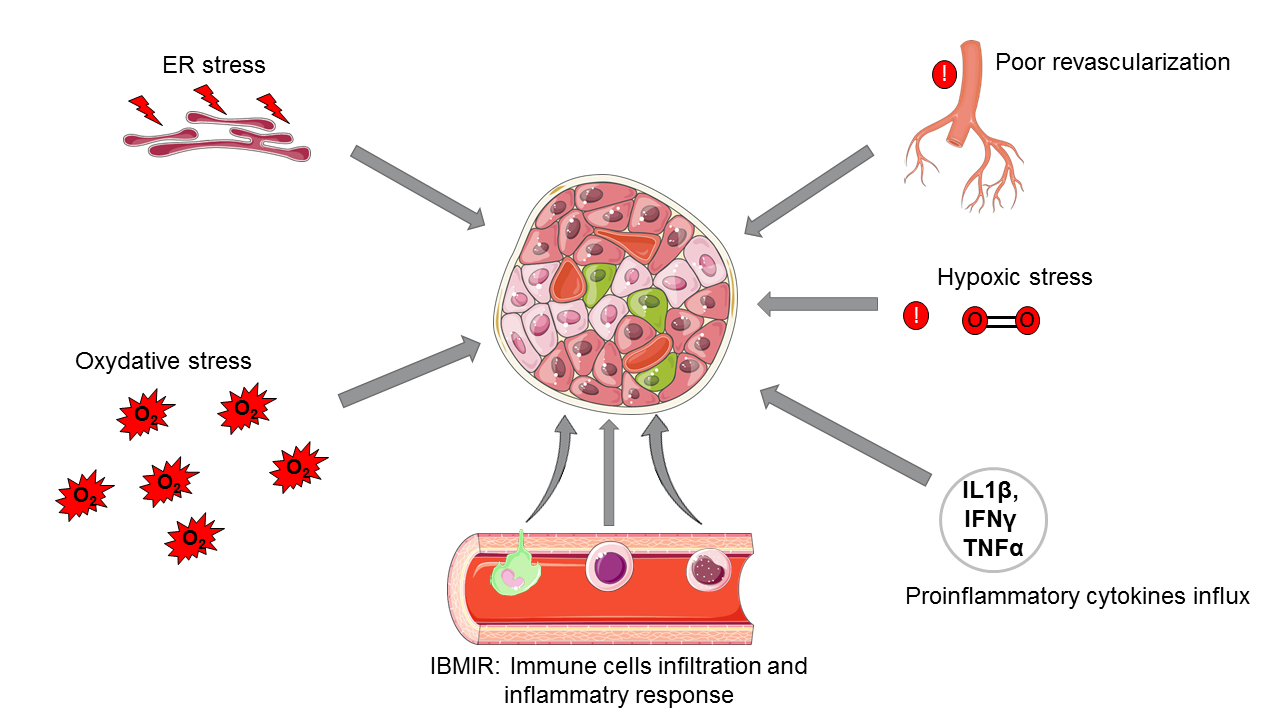
As a result, an average of four pancreas are needed to transplant a patient and improve his glycemicregulation.
Consequently, it is still difficult to respond to the need of all diabetic patients requiring a transplant. Thus, it is important to develop new strategies to improve this therapeutic approach and reduce this excessive number of pancreas to be used. With this in mind, CeeD is interested in myokines, which are molecules secreted by the muscle and regulated by physical exercise [13].
Furthermore, these myokines have been shown to have a bimodal effect on insulin secretion and pancreatic islet survival. Indeed, when they are secreted by healthy skeletal muscle, they positively affect pancreatic islet function and viability with a stimulation of the insulin signaling pathways in β pancreatic cells. Conversely, myokines secreted by insulin-resistant skeletal muscle have a deleterious effect on pancreatic islets [14].
Thus, the objective of the laboratory is to identify the myokines having a beneficial effect on the islets in order to optimize the success of transplantation and to be able to offer it to a greater number of patients with T1D.
Focus on "Exosomes", another CeeD project on cell therapy
To reduce pancreatic islet transplantation complications (such as islet inflammation during isolation, immune rejection, and issues with revascularization post-transplant), the « Exosomes » project seeks to advance islet transplantation by using extracellular vesicles (EVs), which are lipid nanoparticles released by most cells and are essential for intercellular communication. Indeed, extracellular vesicles carry bioactive molecules like lipids, proteins, and nucleic acids, which can influence recipient cells. Thus, this research aims to improve islet viability and function during transplantation while also studying how EVs impact immune cells, such as macrophages. By understanding these interactions, the project hopes to enhance the effectiveness of islet transplantation and better manage type 1 diabetes.
Heiskanen, M. A., Honkala, S. M., Hentilä, J., Ojala, R., Lautamäki, R., Koskensalo, K., Lietzén, M. S., Saunavaara, V., Saunavaara, J., Helmiö, M. et al. (2021). Systemic cross-talk between brain, gut, and peripheral tissues in glucose homeostasis: effects of exercise training (CROSSYS). Exercise training intervention in monozygotic twins discordant for body weight. BMC Sports Science, Medicine & Rehabilitation, 13(1), 16.
Pathak, V., Pathak, N. M., O'Neill, C. L., Guduric-Fuchs, J., & Medina, R. J. (2019). Therapies for type 1 diabetes: current scenario and future perspectives. Clinical medicine insights. Endocrinology and Diabetes, 12, 1179551419844521.
Shapiro, A. M., Lakey, J. R., Ryan, E. A., Korbutt, G. S., Toth, E., Warnock, G. L., Kneteman, N. M., & Rajotte, R. V. (2000). Islet transplantation in seven patients with type 1 diabetes mellitus using a glucocorticoid-free immunosuppressive regimen. The New England Journal of Medicine, 343(4), 230–238.
Abels, E. R., & Breakefield, X. O. (2016). Introduction to extracellular vesicles: biogenesis, RNA cargo selection, content, release, and uptake. Cellular and Molecular Neurobiology, 36(3), 301–312.
Pancreas
As explained
here, type 2 diabetes is characterized by a relative insulin deficiency, of which insulin resistance is an important player. According to datas from the literature and our previous work, several signals originating in particular from the various organs involved in glycaemic regulation (skeletal muscle, liver, adipose tissue, etc.) can induce an alteration of pancreatic beta cells function and probably a decrease in beta cell mass. In this context, our research team was the first to show that communication between insulin-resistant skeletal muscle and pancreatic β cells occurs during the development of diabetes.
Indeed, Dr. Karim BOUZAKRI has shown that insulin-resistant skeletal muscles secrete myokines, which induce a loss of insulin secretion and destruction of pancreatic beta cells. On the contrary, myokines secreted by healthy skeletal muscle tend to have a positive effect on these cells. This is why, at CeeD, our aim is to identify myokines that have a beneficial effect on pancreatic beta cells survival and function in order to develop future treatments for patients with diabetes.
Muscle
Skeletal muscle dysfunction is involved in the development of type 2 diabetes. It is characterized by an inability to respond to insulin (insulin resistance) which lead to a decrease in glucose uptake into the tissue and so participate in chronic hyperglycaemia. Moreover, diabetes is characterized by the decrease of beneficial secretions and the increase in deleterious secretions, such as inflammatory cytokines.
All of these changes participate to the establishment of insulin resistance in other insulin-responsive organs and exhaustion of insulin secretion by pancreatic beta cells. Thus, skeletal muscle dysfunctions are involved in alterations in glucose homeostasis and, on the long term, in the development of type 2 diabetes.
Adipose tissue
As insulin-responsive organ, adipose tissue is involved in the development of type 2 diabetes, particularly in a context of obesity. An imbalance between energy intake and expenditure leads to an increase in free fatty acids (FFA) and glucose uptake in adipose tissue.
The resulting tissue expansion is associated with dysfunctions such as insulin resistance or increase in FFA and inflammatory cytokines release. These secretions can then be deleterious for the functions of adipose tissue but also for other organs (pancreas, skeletal muscle, liver) and contribute to the development of systemic insulin resistance and type 2 diabetes.
Liver
As explained here, the first hallmark of type 2 is insulin resistance. This first characteristic induces in the liver the increase of glycogenolysis and gluconeogenesis, enhancing blood glucose level. This excess of glucose in the blood is usually compensate by adipose tissue and transform to fatty acid, however when the limit of storage is reached, these fatty acids are going to accumulate in the liver. In physiological conditions, compensatory mechanisms are set up by the liver to regulate this lipid accumulation, such as fatty acid β-oxidation [1]. In the case of insulin resistance, in addition to the reduced capacity of the adipose tissue to store fatty acids, the usual hepatic mechanisms to compensate fatty acid accumulation are no more efficient and other mechanisms take place such as an increase of the de-novo lipogenesis linked to the overexpression of factors such as SREBP1c [2]. Thus, hepatic insulin resistance induces hepatic lipid accumulation, which is known as the first hit of Metabolic Dysfunction-Associated Steatotic Liver Disease (MASLD, formerly known as NAFLD) [1].
MASLD is the most common liver chronic disease with a worldwide prevalence of 25% [3]. Among NAFLD patients, 25% will progress towards advanced form of the disease, such as a metabolic dysfunction-associated steatohepatitis (MASH, previously known as NASH) [3]. MASH, in addition to lipid accumulation, involves hepatocellular damages, oxidative stress, inflammation and fibrosis that can progress to cirrhosis, also known as the second hit of the disease [1]. Finally, the most advanced stage of the disease is the hepatocellular carcinoma (HCC), for which the only solution is transplantation.
To date, no specific treatment of MASH exists [3], [4]. The first recommendation is also hygieno-dietary measures comprising physical exercise. Thus, the objective of this project is to develop a therapeutic strategy to treat MASH. Our hypothesis is that myokines could present potential beneficial effects on different mechanisms involved in MASH development.
Kidney
In diabetes conditions, glucose reabsorption in the blood circulation is increased of about 10% contributing to hyperglycaemia [5], indeed the threshold of reabsorption of the kidneys’ glucose transporters is higher in diabetic patients compared to non-diabetic persons. In consequences, the glomerulus become damaged and do no more filtrate the plasma efficiently et many proteins are eliminated in the urine, notably the albumin [5]. The beginning of albumin presence in the urine, called micro-albuminuria, is known as the first hit of diabetic nephropathy. 20 to 40% of diabetic patients with microalbuminuria progress to severe form of nephropathy, such as macro-albuminuria , the decrease of the glomerular filtration rate, the increase of blood pressure and the increase of the cardiovascular morbidity and mortality [5].
The main characteristics of diabetic nephropathy are: glomerular damages, nodular mesangial expansion and the thickening of the glomerular membrane [6]. Extra-glomerular damages are also present, such as tubular atrophy, inflammation and fibrosis linked to the loss of renal function [6].
Today, nephropathy diagnosis is carried out upon diabetes diagnosis [7]. To date, the main treatments are anti-diabetics and blood pressure control, which only slow down the nephropathy progression, hence no specific treatment exists [7].
Impact of the muscle secretome on diabetes-related metabolic diseases
As explained here, the muscle as a real impact on insulin and glucose homeostasis, mostly through the impact of its secretome on the different insulin-sensitive organs. Furthermore, it has been highlighted that its secretome’s content is dependent on the patients’ status: healthy or diabetic. Preliminary datas (unpublished) from the laboratory have highlighted beneficial impact of some muscle products on pancreatic β cells and notably on their survival, but also on different cell mechanisms also involved in the different diabetes-related diseases. Thus, our hypothesis is that this muscle’s secretome could also have beneficial effects on the different diabetes-related complications studied at the laboratory. For that, the aim of our laboratory’s research is to identify and highlight product of the muscle such as myokines or exosomes that could have beneficial impact on diabetes and its complications.
[1] M. Noureddin et A. J. Sanyal, « Pathogenesis of NASH: the Impact of Multiple Pathways », Curr Hepatology Rep, vol. 17, no 4, p. 350‑360, déc. 2018, doi: 10.1007/s11901-018-0425-7.
[2] V. Azzimato et al., « Liver macrophages inhibit the endogenous antioxidant response in obesity-associated insulin resistance », Sci. Transl. Med., vol. 12, no 532, p. eaaw9709, févr. 2020, doi: 10.1126/scitranslmed.aaw9709.
[3] A. Majumdar, « Non-alcoholic fatty liver disease: Current therapeutic options », Current Opinion in Pharmacology, p. 8, 2021.
[4] N. Chalasani et al., « The Diagnosis and Management of Non-alcoholic Fatty Liver Disease: Practice Guideline by the American Gastroenterological Association, American Association for the Study of Liver Diseases, and American College of Gastroenterology », Gastroenterology, vol. 142, no 7, p. 1592‑1609, juin 2012, doi: 10.1053/j.gastro.2012.04.001.
[5] P. Curtis L. Triplitt CDE, « Understanding the Kidneys’ Role in Blood Glucose Regulation », Supplements and Featured Publications, vol. 18, no 1 Suppl, avr. 2012, Consulté le: 10 mars 2022. [En ligne]. Disponible sur: https://www.ajmc.com/view/ace005_12jan_triplitt_s11
[6] C. Qi, X. Mao, Z. Zhang, et H. Wu, « Classification and Differential Diagnosis of Diabetic Nephropathy », Journal of Diabetes Research, vol. 2017, p. 1‑7, 2017, doi: 10.1155/2017/8637138.
[7] N. Samsu, « Diabetic Nephropathy: Challenges in Pathogenesis, Diagnosis, and Treatment », BioMed Research International, vol. 2021, p. 1‑17, juill. 2021, doi: 10.1155/2021/1497449.
[8] A. M. J. Shapiro et al., « Islet Transplantation in Seven Patients with Type 1 Diabetes Mellitus Using a Glucocorticoid-Free Immunosuppressive Regimen », N Engl J Med, vol. 343, no 4, p. 230‑238, juill. 2000, doi: 10.1056/NEJM200007273430401.
[9] A. M. J. Shapiro, M. Pokrywczynska, et C. Ricordi, « Clinical pancreatic islet transplantation », Nat Rev Endocrinol, vol. 13, no 5, p. 268‑277, mai 2017, doi: 10.1038/nrendo.2016.178.
[10] D. J. Holmes-Walker et al., « Islet Transplantation Provides Superior Glycemic Control With Less Hypoglycemia Compared With Continuous Subcutaneous Insulin Infusion or Multiple Daily Insulin Injections », Transplantation, vol. 101, no 6, p. 1268‑1275, juin 2017, doi: 10.1097/TP.0000000000001381.
[11] K. Verhoeff, B. A. Marfil-Garza, et A. M. J. Shapiro, « Update on islet cell transplantation », Current Opinion in Organ Transplantation, vol. Publish Ahead of Print, juin 2021, doi: 10.1097/MOT.0000000000000891.
[12] R. Bottino, M. F. Knoll, C. A. Knoll, S. Bertera, et M. M. Trucco, « The Future of Islet Transplantation Is Now », Front. Med., vol. 5, p. 202, juill. 2018, doi: 10.3389/fmed.2018.00202.
[13] M. A. Febbraio et al., « Glucose Ingestion Attenuates Interleukin‐6 Release from Contracting Skeletal Muscle in Humans », The Journal of Physiology, vol. 549, no 2, p. 607‑612, juin 2003, doi: 10.1113/jphysiol.2003.042374.
[14] K. Bouzakri, P. Plomgaard, T. Berney, M. Y. Donath, B. K. Pedersen, et P. A. Halban, « Bimodal Effect on Pancreatic β-Cells of Secretory Products From Normal or Insulin-Resistant Human Skeletal Muscle », Diabetes, vol. 60, no 4, p. 1111‑1121, avr. 2011, doi: 10.2337/db10-1178.